#17 - Composites in Plain English Pt. 1
For my first few projects, I’ll be messing around with composites. I’ve had a fair amount of experience working with composites, but I still feel like I don’t have an intuitive understanding of them. Seeing how composites are used in practice, I suspect that’s true for other engineers as well. There’s a joke about “black aluminum” made when engineers use carbon fiber in a way that does it no justice. I think our schools and books are partly responsible -- there’s usually an abrupt jump from “what is a composite” to “let’s calculate ABD matrices.” I hope to improve my (and your) understanding of them by talking through the basic concepts in plain English.
What Is a Composite?
A composite is simply a combination of two or more different materials. Nowadays, composites are associated with just a small subset of materials, like fiberglass and carbon fiber, which have only been around for the last century or so. But composites are not a new technology. Man-made composites have been around since at least ancient Egypt, for making bricks (using a combination of straw and mud) and making mummy cases and masks (using papyrus and plaster, a precursor to papier-mâché). If we look to natural materials, wood is itself a composite of lignin and cellulose. In general, the aim of any composite is to combine materials in a way that is greater than the sum of its parts.
So why is something like steel not considered a composite - even though it’s a combination of two different materials, iron and carbon? The difference is that, after mixing, the individual ingredients in steel don’t remain distinct like they do in composites. It’s like the difference between chocolate chip ice cream (chocolate and ice cream keep their separate identities) and chocolate flavored ice cream (chocolate and ice cream become one).
Composites as a whole are much too big of a topic to talk about, so I’ll be focusing here on the modern fiber-based materials, like carbon fiber. If you haven’t seen a carbon fiber part being made, the process is very similar to the papier-mâché project many of us did in art class. (My own brilliant childhood piece is shown below - hopefully it doesn’t give you nightmares.) We took strips of paper, wet them with glue, and put them over a balloon. When the glue dried and hardened, we popped the balloon to release the mask. The process of making carbon fiber is pretty similar. The carbon fiber in cloth form (like the paper strips) is wet with resin (a fancy glue) and placed into a mold (the balloon) until hard.
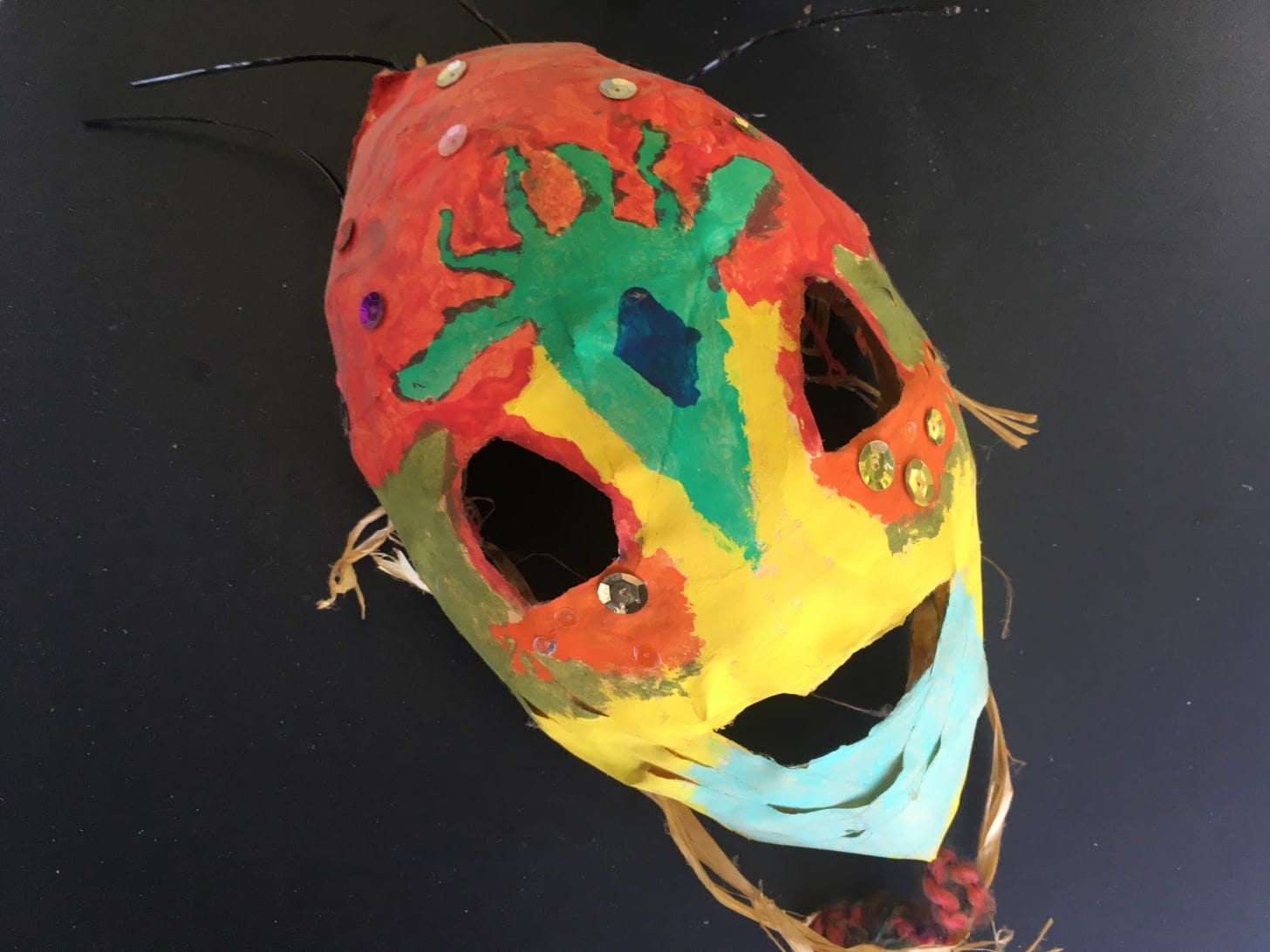
The Fiber
Modern composites follow the pattern described above. There’s a cloth and there’s a resin. The cloth used in composites is not so different from the one in your shirt. Both are made of yarns woven together. The yarn itself is a long string of fibers. The only difference is that, in your shirt, the fiber is probably cotton. In composites, the fiber is generally glass or carbon. Hence fiberglass and carbon fiber.
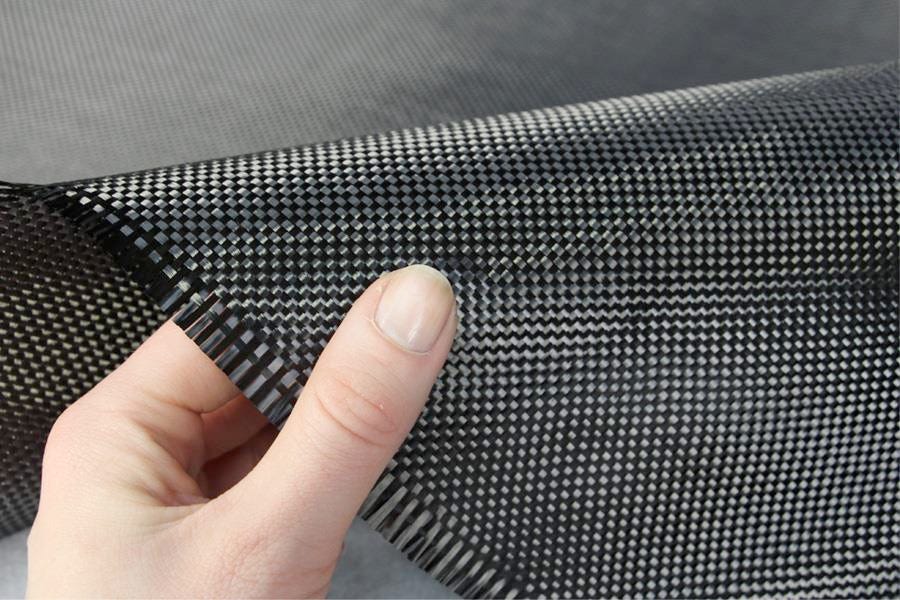
(Not my hand pictured above)
So what’s the deal with everything being a fiber? Wouldn’t it be easier to make parts out of solid glass rather than to go through the trouble of making thin fibers of glass, a cloth out of those fibers, and finally a part out of that fiberglass cloth? It’s the same material whether it’s in a glass jar or in a length of fiberglass cloth, isn’t it? To understand why it’s important to go through all that trouble, we have to learn a little bit about how things break.
You’ve probably struggled to tear open a bag of chips before. Then after struggling a bit, you notice the “tear here” notch or use your canines to make a notch of your own like an absolute animal. When you pull apart at the notch, the bag opens relatively easy. The notch, in engineering circles, is more generally called a stress concentration. As soon as you have something like a crack, a notch, or a sharp corner, stresses (i.e., internal forces) concentrate there and it doesn’t take much effort to continue it across. (This is why engineers like rounding everything off.) In other words, it’s way easier to propagate an existing crack than to start a new one.
It turns out that the same issue exists at the molecular level. If a material’s strength was based solely on the amount of energy it takes to drive two layers of atoms apart, things would be ridiculously strong. Steel should theoretically be ten times stronger than it is. But in reality, we don’t get close to that because of minuscule defects or “notches” on the surface of the material.
In a fiber, there are also defects, but since the fiber itself is thinner than a human hair, the defects are smaller too. You can’t have a .001” wide scratch on something that’s only .0005” wide -- the scratch can’t be bigger than the fiber itself. And smaller defects are proportionally harder to open up. In glass, micro scratches will appear on a surface simply by handling the material, so it happens naturally during manufacturing and not necessarily because someone took a key to it. Thinner glass fibers may also get scratched less as they’re handled because they’re more flexible and can bend out of the way. As you can see below, as a result, thinness has a huge positive effect on strength.
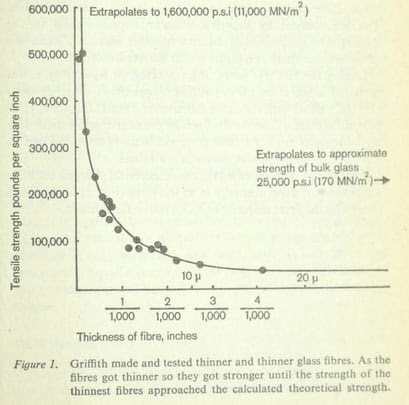
Interestingly, the same phenomenon occurs even in materials, like carbon, that don’t scratch as easily as glass. Inherently, there are irregularities (i.e., small steps) at a molecular level on the surface of a material. You can think of it as stacking Pez, with Pez as the molecules in a material. In a small diameter Pez dispenser, it’s easier to keep the Pez pretty well aligned. This represents the material in the form of a thin fiber. In a larger diameter jar, you’ll have more irregularities where the Pez just kind of form a pile and don’t line up neatly. This represents the material in a larger, “bulk” form. In this bulk form, the steps on the surface (the side of the Pez stack) are larger. The larger those steps, the higher the stress concentration, the weaker the material.
The other benefit of the thinner fiber is its internal molecular structure. Turning back to the Pez analogy, the Pez in the dispenser are well-aligned in an unbroken chain. The Pez in the jar, when stacked neatly, will have those same well-aligned sections. But those sections will be interrupted by rogue Pez that have flipped sideways or groups that have branched off in a different direction. In the dispenser, a higher percentage of the Pez are aligned continuously in the desired orientation. The same is true of our fiber. A higher percentage of the molecules are aligned in the desired orientation, all pulling in the same direction, giving us a higher strength to weight ratio.
All that to say, the reason thinner fibers are important to composites is because they’re stronger! To clarify, when I say strength, it’s relative to the thickness. I’m not saying that a thin fiber will support more weight than a thick one. But if you had a bundle of thin fibers that together matched the size of the thick one, they absolutely would. That’s why it’s worth going through all the extra effort to make fibers, yarn, and fiberglass cloth before making a part. Your part will be stronger than if you made it from solid glass.
Admittedly, this atomic-level stuff is not really my strong suit (stinks a little too much of chemistry). If you’d like to read more, the chart and much of this knowledge is from J.E. Gordon’s The New Science of Strong Materials.
This post has gotten a little long, so I’ll end it here. In the next one, I’ll talk about the glue and how glue and fiber work together.
Questions, corrections, clarifications? I’d love to have your input.
Drawing exercise #7. If you missed it, here’s why I’m learning to draw.
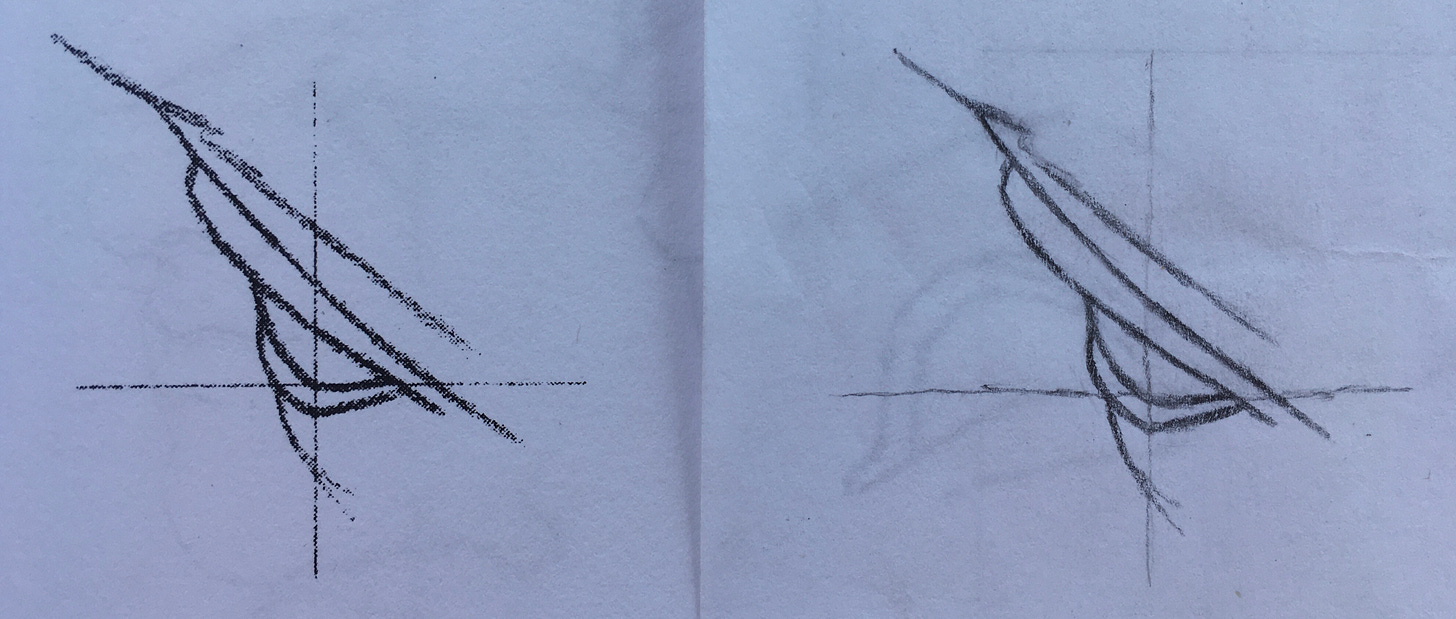